The Battle of Probiotics and Their Derivatives Against Biofilms
Authors: Abolfazl Barzegari, Keyvan Kheyrolahzadeh, Seyed Mahdi Hosseiniyan Khatibi, Simin Sharifi, Mohammad Yousef Memar, and Sepideh Zununi Vahed
Published: 2020 Feb 26
Abstract
Biofilm-related infections have been a major clinical problem and include chronic infections, device-related infections and malfunction of medical devices. Since biofilms are not fully available for the human immune system and antibiotics, they are difficult to eradicate and control; therefore, imposing a global threat to human health. There have been avenues to tackle biofilms largely based on the disruption of their adhesion and maturation. Nowadays, the use of probiotics and their derivatives has gained a growing interest in battling against pathogenic biofilms. In the present review, we have a close look at probiotics with the ultimate objective of inhibiting biofilm formation and maturation. Overall, insights into the mechanisms by which probiotics and their derivatives can be used in the management of biofilm infections would be warranted.
Keywords: antibiotic resistance, biofilm, probiotics, lactobacillus, sepsis, infection
Introduction
Biofilms are the aggregates of micro-organisms that are embedded in a self-produced polymeric matrix in a sessile state.1 In the history of microbiology, biofilms have been detected earlier; however, only recently has their clinical burden been fully recognized. According to the National Institutes of Health (NIH), biofilms are involved in approximately 65% and 80% of all microbial and chronic infections, respectively. In the clinic, microbial biofilms through colonization on implants (prosthetic heart valves, catheters and joint replacement) and medical devices, account for hospital-acquired infections that make the patients easily infected by certain pathogens. Moreover, biofilm infections lead to different disorders, for instance, diabetes mellitus, dental caries, medical implants and wound infections that significantly affect the quality of life, cancer development, and subsequently, increase the global morbidity rate.1
Hardly are biofilms detectable with routine diagnostic tests; therefore, the management of their infections are challenging in the clinic.2 Methicillin-resistant Staphylococcus aureus (MRSA), Streptococcus mutans, Pseudomonas aeruginosa, S. epidermidis and Gardnerella vaginalis are the most common biofilm formers in the clinic.3 Different strategies like new generations of antibiotics and the inhibition of biofilm formation by quorum sensing (QS) inhibitors have been developed. Due to the challenges of these therapeutic agents in the clinic, there is a demand for developing new strategies. Recent evidence indicates that one of the strongest options for fighting pathogenic biofilms would be probiotics.
Probiotics are living bacteria that confer a health-related profit to the host when administered in acceptable doses. This action of probiotics is mediated by interacting with host gut microbiota. High-throughput approaches including transcriptomics, metabolomics, proteomics and metagenomics have revealed that probiotics present beneficial for the host and they can modify host mucosal and systemic immune responses and protect the host against pathogens.4 Lactobacillus (lactic Acid Bacteria, LAB) and Bifidobacterium are the most important microbial genera that are generally used in the preparations of probiotics. These strains support a balanced immune function, healthy gut microbiome and improved nutrient absorption and lead to a healthy host.5 They are also capable to potentially modulate the microbial ecology of biofilms by pathogens’ growth inhibition, adhesion and co-aggregation. Furthermore, probiotics exert antimicrobial activities against the gastrointestinal (GI) tract pathogens via declining luminal pH, competing for adhesion sites and nutrients and producing antimicrobial agents such as bacteriocins, hydrogen peroxide and organic acids (Tables 1 and and2).2). Based on these properties, probiotics present effectiveness in managing biofilms.
In this review, first, we have an overview on the mechanisms of biofilms formation and approaches for combating biofilms. Then, we highlight the novel probiotic-based progressive strategy to manage the pathogenic biofilms with emphasizing on probiotics’ molecular mechanisms of actions.
Biofilm Formation
A biofilm is an agglomeration of micro-organisms on biotic and abiotic substances.7 The formation of biofilm is not accidently, it is programmed with a complex mechanisms, whereby their lifecycle involves different distinct stages, from bacterial attachment and adherence to maturation and the release of cells from the matrix7,8 (Figure 1). Beyond guarding the bacterial cells, biofilms ease the distribution of antibiotic resistance via stimulating horizontal gene transfer.9 In the course of biofilm formation, various bacterial species display social behaviors and communicate with each other through a quorum sensing (QS) mechanism.10
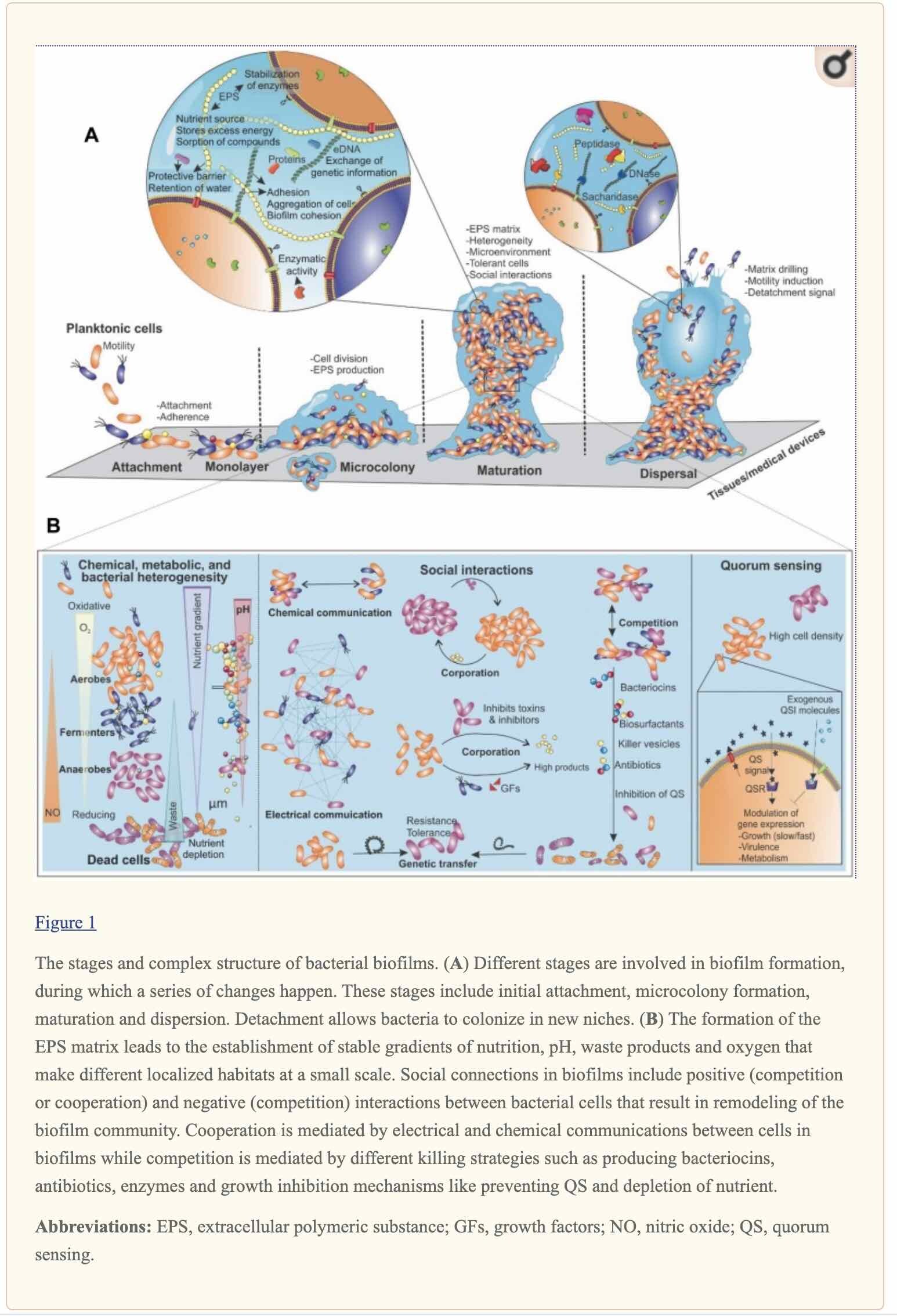
QS is a bacterial cell-to-cell communication that regulates gene expression coordination and detection of cellular density that is mediated by hormone-like small organic compounds called auto-inducers (AIs). Using these signaling molecules, bacteria collectively regulate the expression of virulence factors, the production of secondary metabolite, biofilm development and communications with host and other microbes based on population density. During the process of QS, signaling molecules bind to new bacterial receptors and lead to the transcription of genes within a single bacterial species and between different bacterial species that enable intraspecies and interspecies communications.
Treatment Strategies for Combating Bacterial Biofilm Infections
Currently, biofilm infection therapy is a complex challenge for clinicians. Antibiotic treatment is insufficient in combating against biofilm-related infections; however, understanding the nature of biofilms helps us support our efforts to fight with biofilm infections. Biofilm treatment can include the elimination of infected foreign bodies, the choice of well-penetrating and sensitive antibiotics, early administration of high dosage antibiotics/combinations and the usage of biofilm dispersal and/or anti-QS agents.11 In the following sections, we have a brief view of the biofilm-battling strategies, then a close look at the impacts of probiotics and their derivatives on biofilms will be discussed.
Prescribing Antibiotics
Different antibiotics like lincosamides, rifamycins, tetracyclines, macrolides, etc. penetrate better than β-lactam, glycopeptides, aminoglycosides and polymyxin into the cells and tissues.11 The combination therapy of antibiotics also is better than antibiotic monotherapy against biofilm infection. Beyond the proper selection of antibiotics, appropriate duration of antibiotic treatment is essential. Despite a superior ability of fluoroquinolones for Gram-negative bacteria and rifampicin for Gram-positive bacteria to counteract biofilms, the entire eradication of biofilm infection is still challenging. Antimicrobial agents used for treatment of infections are not effective on biofilm forming bacteria, since they induce a selective pressure on the pathogens which triggers development of resistance to certain agents.
Suppressing of Quorum Sensing
Targeting the Quorum sensing mechanisms has been a striking strategy to control infection in which bacterial virulence is attenuating to be easily cleared by the host immune system and not to establish an effective infection. Quorum sensing inhibitory compounds are a new generation of antimicrobial agents; however, they have not been largely successful. Several strategies are available to disturb bacterial QS. One of these strategies is the inactivation of LuxR homologs using N-acyl homoserine lactone (AHL) antagonists that competes with the native AHL to bind to the LuxR-type receptor. By inhibiting AHL-binding, the LuxR homolog would not be activated and the expression of virulence factor gets switched off. The suppression of the AHL synthesis is yet another strategy.15 While QS suppression has been studied as novel anti–infective strategy, evidence shows the development of bacterial resistance against QS-suppressing agents.16 In addition, the toxicity of some QS- suppressing compounds, such as nanoparticles, limits their biomedical usage.
Probiotics Fight Against Biofilm Formation
Due to the insufficiency of well-known approaches, the development of novel biofilm-fighting strategies would be valuable in the clinic. Recent evidence indicates that probiotics have opened a new horizon to fight with infectious biofilms. Since probiotics cannot induce the strong selective pressure on resistant isolates than conventional antibiotics and also they are less cytotoxic than QS-suppressing agents, they can be considered as ideal option for new anti–virulence agents. Using different mechanisms, probiotics can hinder the activity of pathogenic bacteria and their adhesion to surfaces. Moreover, they prevent QS, biofilm formation and the survival of biofilm pathogens, interfere with biofilm integrity/quality and finally lead to biofilm eradication
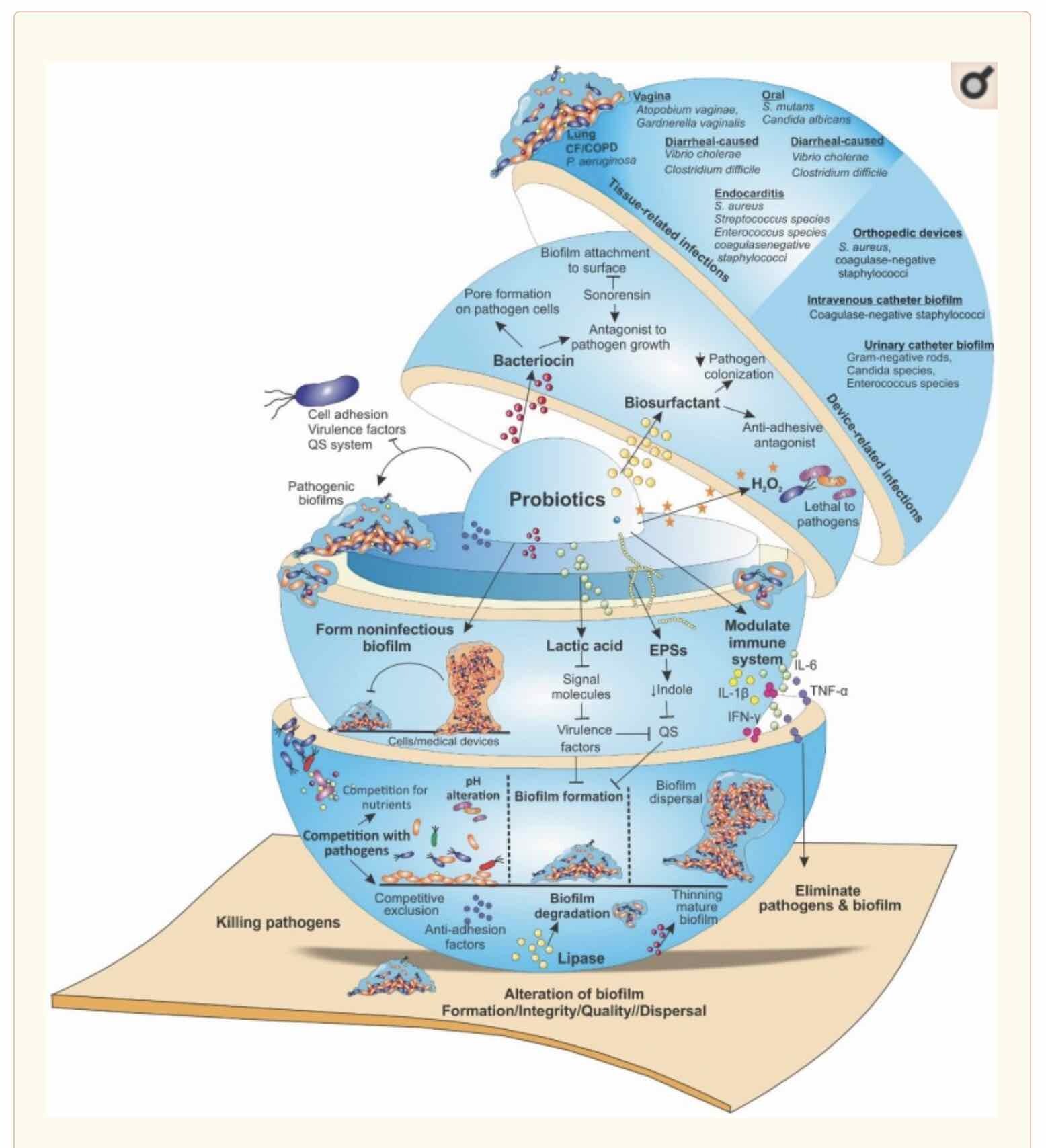
Targeting microbial biofilms by probiotics. Probiotics employ different mechanisms by which interfere with the activity of pathogenic bacteria. They produce antagonistic substances such as, surfactants, bacteriocins, EPS, organic acids, lactic acid, fatty acids, enzymes (lipase, amylase) and hydrogen peroxide that can hinder the activity of pathogenic bacteria and their adhesion to surfaces. Moreover, they prevent QS, biofilm formation and the survival of pathogens as well as interfere with biofilm integrity/quality, finally, lead to biofilm eradication. Furthermore, probiotics generate unfavorable environmental conditions for pathogens (e.g., pH alteration as well as competition for surface and nutrients). Their competitive adhesion to human tissues or medical devices (catheters, prostheses, or other medical devices), prevent the colonization of harmful bacteria. Additionally, by modulating host immune responses and formation of non-pathogenic biofilms, they target pathogenic biofilms that prevent the biofilms formation by certain pathogenic bacteria.
Abbreviations: CF, cystic fibrosis; COPD, chronic obstructive pulmonary disease; EPS, extracellular polymeric substance; QS, quorum sensing; UTI, urinary tract infection.
The probiotic strains can be isolated from numerous sources such as human, animal, plant, environment and foods.18,19 Then, they can be identified and characterized by microbiological, biochemical and molecular-based techniques. Streptococcus salivarius, S. oralis, L. rhamnosus, L. fermentum, L. plantarum L. casei, L. acidophilus, L. brevis, L. sporogenes, L. salivarius, L. delbrueckii, L. pentosus, Bifidobacterium lactis and B. longum are the most reported probiotic strains that exert anti-biofilm activity (Tables 1 and and22).
Several in vitro biofilm models have been developed by attaching bacteria on adhesive surfaces.20 All of these models lack features of the host immune competence and environment. So, animal models take into account since it is practically impossible to study the development of infectious diseases in humans (reviewed comprehensively in Ref [21]). MRSA mouse model22 and rabbit model of ischaemic and infected wounds were developed. Moreover, a removable in vivo abutments was developed that mimicked dental implants. To address in vitro and in vivo problems, a novel human plasma biofilm model was developed for studding the impact of probiotics on pathogens that mimicked a biofilm-challenged human wound milieu.
Probiotic Products Against the Different Pathogenic Biofilms
Lactobacillus species produce different exometabolites such as EPSs, bacteriocins, oxygen reactive species (ROS) and biosurfactants with anti-biofilm activity. The polysaccharides produced by LAB possess anti-biofilm, immune system stimulatory and antioxidant effects. The EPS of Lactobacillus spp. was effective in both Gram-positive (e.g., Listeria monocytogenes and S. aureus) and Gram-negative (e.g., P. aeruginosa and Salmonella typhymurium) bacteria. The results displayed that the biofilm removal ability is related to EPS concentration.
The anti-biofilm activity of bacteriocins has been demonstrated in different reports. L. brevis DF01 bacteriocin prevents biofilm formation but does not eradicate the established Escherichia coli and S. typhimurium biofilms.32 The mechanisms of biofilm inhibitory effects of bacteriocin are not well understood. Some of the bacteriocins eradicate biofilm by the induction of pore-formation on the bacterial cell surface, leading to ATP efflux, while some others have biological activity by proteolytic enzymes.33 Subtilosin A, a cyclic bacteriocin (lantibiotic protein) synthesized by Bacillus subtilis, is another derivative of probiotics. It has a net cationic charge that generally targets the surface receptors rather than binding to bacterial cells electrostatically. Beside the antimicrobial activity of subtilosin against Gardnerella vaginalis and L. monocytogenes, its anti-biofilm effect was reported against G. vaginalis alone and with natural antimicrobial agents. Given the wide-ranging activities of subtilosin, Chikindas et al observed its anti-QS effect in E. coli O157:H7, L. monocytogenes ScottA and G. vaginalis ATCC 14018. Subtilosin led to the inhibition of 60% of E. coli, 80% of L. monocytogenes and 90% of G. vaginalis biofilms.37 Likewise, sonorensin, a bacteriocin produced by Bacillus sonorensis MT, was able to decrease S. aureus biofilms cell viability, inhibit biofilm attachment and formation, and cause the thinning of mature biofilms.
Due to exometabolites formation, Lactobacillus species also inhibit Candida albicans biofilm by inhibiting the initial stage of colonization and hypha formation.39 Lactobacilli that produce biosurfactant had antimicrobial, anti-adhesive properties and aggregation ability against pathogenic biofilm formation.40 L. rhamnosus producing biosurfactants could disrupt the physical membrane structure or protein conformations; resulting in cell lysis.41 Furthermore, biosurfactants significantly decrease the adhesion and biofilm generation of bacteria in a dose-dependent manner.28
Probiotics Influence Gene Expression of Pathogenic Biofilms
The mechanism by which probiotics prevent the biofilms formation is fairly unclear. Several in vitro studies have shown that the expression of genes involved in cell adhesion, QS, virulence factors and biofilm formation can be influenced by probiotics. Wasfi and coworkers assessed the Lactobacillus spp. effect on the gene expression of S. mutans in a co-cultured condition. They focused on genes involved in EPSs formation (gtfB, sacB (ftf), gtfC and gtfD), signal transduction systems (vicR, comC, vicK and comD) and stress survival (atpD and aguD). Results revealed that there was an overall significant decrease in the expression of these genes among different groups, in both biofilm-forming and planktonic cells. Additionally, by producing organic acid and peroxide, probiotics led to a decline in cell adherence and preformed biofilm. Moreover, EPS produced by L. acidophilus A4 considerably could inhibit biofilm formation of E. coli O157: H7 by reducing the expression of genes related to chemotaxis (cheY) and curli formation (csgA, csgB and crl).29 Burton et al clarified a mechanism of biofilm inhibition of C. albicans using the combination of L. plantarum SD5870, Streptococcus salivarius DSM 14685 and L. helveticus CBS N116411. The expression of some C. albicans genes such as ALS3 (adhesin/invasin), HWP1 (a critical hyphal wall protein for biofilm formation), EFG1 (hyphae-specific gene activator) and SAP5 (secreted protease) are affected by these probiotics. The results showed that these probiotics are effective in inhibiting the biofilm formation and also removing of the preformed biofilms of C. albicans. Therefore, it is rational to claim that probiotics and their derivatives can be used as both prophylactic and treatment biodrugs.
Some probiotics have also inhibitory effects on QS systems that inhibit the QS-dependent physiologic behaviors of bacteria.44 Lactic acid produced by probiotics had shown an inhibitory effect on QS by suppressing short-chain AHL production and biofilm formation of P. aeruginosa that is regulated by QS.44 Probiotics also secret organic acid as QS antagonists that interfere with AHLs production at the gene expression level and prevent biofilm formation.45
Biosurfactants isolated from L. plantarum and Pediococcus acidilactici could inhibit the adhesion and biofilm formation of S.aureus CMCC 26003 in a dose-dependent manner in vitro. The molecular mechanism of biosurfactants is mediated by affecting the expression of biofilm-related (cidA, sarA, icaA, dltB, sortaseA, and agrA) genes and interfering with the release of signaling molecules (AI-2) in QS systems.28 Similarly, S. mutans produce extracellular glucans by glucosyltransferases (gtfs) that are vital for the initiation and progression of dental caries. Biosurfactant produced by L. fermentum could decrease the S. mutans gtfB/C gene expression, the process of attachment and biofilm formation.
Probiotics Modulate the Host Immune Responses to the Biofilms
The host immune responses against biofilms are mediated by various cellular receptors, chemokine and cytokine expression, that can be different based on the stage of biofilm. Probiotics and their secreted soluble factors are speculated to be recognized by the toll-like receptors (TLRs) on epithelial cells; and thereafter exert their immunomodulatory effects on intestinal and systemic immunities.48 Moreover, probiotics can modify innate immune functionality in different ways, some of which include the secretion of immunomodulatory metabolites, lipids and proteins, receptor expression, micro-RNAs induction and production of negative regulatory signaling molecules. Therefore, by modulating the immune responses, probiotics can impact biofilms indirectly. Streptococcus thermophilus strains (ST1342, ST1275, and ST285) can activate monocyte cells to secret IL-1β, TNFα, IL-6 and IFN-γ that activate the innate immune responses in order to eliminate pathogens. Strain ST1342 could induce high levels of IL-1β secretion that has both anti–viral and anti-bacterial activities.50 Likewise, it was mentioned that the probiotic L. paracasei DG utilized generally in commercial probiotic products, possess immune-stimulatory activities by enhancing of TNFα, IL-6 and CCL20 expression in the human monocyte leukemia cell line.51 Lactobacillus sp. could induce IFN‐γ production and inhibit IL‐10 production and exert immunomodulatory effect on S. mutans in human-cultured cells. Detailed knowledge of the immune mechanisms, the cytokine and receptor expression profiles and bacterial defense mechanisms under biofilm formation is needed for demonstrating the effects of probiotics on the immune system to fight against microbial biofilm.
Probiotic Biofilms Against Pathogenic Biofilm
The formation of biofilm by probiotics is considered to be a beneficial strategy against pathogenic biofilms since they compete with pathogens for nutrients and space with different mechanisms of action. Moreover, probiotic biofilms can stimulate the colonization and longer stability of probiotics in the host mucosa that prohibit colonization of pathogenic bacteria. Only some of Lactobacillus strains such as L. reuteri, L. rhamnosus, L. fermentum and L. plantarum can form biofilm on abiotic surfaces (glass or polystyrene). The EPS production by some biofilm-former probiotics can prevent the biofilms formation of certain pathogenic bacteria.
In line with this subject, Gómez and coworkers tested the protective effect of biofilms with bacteriocinogenic (L. curvatus MBSa3, L. sakei MBSa1, L. lactis VB94 and L. lactis VB69) and non-bacteriocinogenic (Weissela viridescens 113, L. helveticus 354, L. lactis 368, and L. casei 40) lactic acid bacteria to fight against E. coli O157:H7, Salmonella typhimurium and L. monocytogenes. Results show a prevention in biofilm formation of these pathogenic bacteria in 24, 48 and 72h of exposure.60 Moreover, biofilms of probiotic E. coli Nissle 1917 on silicone substrates could decrease the colonization of the pathogenic E. faecalis 210.61 Likewise, L. kunkeei biofilm reduces the infection of P. aeruginosa by affecting biofilm formation and/or their stability.62 Furthermore, biofilms of probiotic formed by Bifidobacterium infantis and L. reuteri can be utilized as efficient bacteria to delay the L. monocytogenes growth.18
L. brevis 104/37, L. plantarum 118/37 and 6E could effectively eradicate staphylococcal biofilms. Yet, only L. rhamnosus ATCC 7469 and L. plantarum 2/37 could form their own biofilms to replace with the pathogenic ones. Additionally, the L. plantarum WCFS1 and NA7 biofilms produce extracellular molecules with immunomodulatory and growth inhibitory properties against food pathogens (S. aureus, E. coli O157:H7, L. monocytogenes, and Salmonella enterica). All the studied Lactobacillus strains had an anti–inflammatory effect in the in vitro, while just L. fermentum NA4 displayed a protective effect in vivo. Hence, Lactobacillus in biofilm status exerts beneficial probiotic properties in a strain-dependent manner.64 The progress of the new technologies for the encapsulation of biofilms that covers in the double coated capsules has developed a new generation of probiotics. L. rhamnosus GG microcapsules, as effective inhibitors of transcriptional activators of the luxS QS system, could prevent biofilm formation and disturb the mature biofilms.
Future Perspective
Biofilm infection therapy has been a complex challenge for clinicians. Better understanding and hacking into bacterial biofilms help scientists develop robust strategies. Recently, the immune system and probiotics relationships have been reported in defending the host against the colonization of pathogenic species. In fact, probiotics yield different compounds, ranging from peroxides and fatty acids to highly specific bacteriocins, to kill or hinder pathogenic bacteria. Recently, clinical trials and in vitro studies have provided evidence on the impact of the probiotics on different medical fields (wound, oral, intestinal and vaginal infections) to fight against pathogenic biofilms via a counteraction, competition and gene silencing of pathogenic factors. All data together signify a great ability of probiotics to be used both in prevention and treatment of pathogenic biofilm infections.
In fact, in vitro studies on adhesion, the secretion of extracellular anti-biofilm factors, metabolic activity, the growth inhibition, co-aggregation, the prevention of biofilm formation and the eradication of mature biofilm have recommended possible roles for probiotic in modifying the biofilms microbial ecology. On the other hand, biofilm-forming probiotic strains can exchange resident biofilm pathogens with a non-pathogenic variant that produce bacteriocin;40 however, their molecular mechanisms have been poorly examined.
Challenges with the Management of Biofilms by Probiotics
Data demonstrate that probiotics and their derived-products can be hopeful strategy to manage biofilms. It should be noticed that data are still scarce and there is not enough evidence to consider probiotics as bio-drugs to inhibit pathogenic biofilm formation and/or disperse pre-formed biofilms. Confounding results may be related to the diversity in delivery vehicle, dose, assessment of efficacy and viability, and particularly to the variability in selection of strains. It has been revealed that the impacts of probiotics are strains-specific, different strains of even one probiotic species can present an altered impact on the host and pathogenic biofilm since the host molecular signaling reprogramming extremely tend to depend on the bacterial strain and cell context. No two probiotics look like each other and different strains may exert different effects. Additionally, under various circumstances, even the same strains may function differently. Therefore, an ideal strain of probiotic for interfering and competing with pathogenic biofilms should be screened and identified at the molecular level for specific pathophysiological states, particularly in the context of definite infection and microbial targets.
Additionally, characterization and evaluation of safety aspects (blood hemolytic activity and resistance to antibiotics) of strains should be performed before their clinical administration. The essential criteria for selection of potential probiotic strains are proposed to be their adhesion to epithelial cells and mucus along with their co-aggregation with pathogens.66 Furthermore, other criteria including potential antimicrobial activity against pathogens, survival in the human GI conditions and inhibition of colon cancer define a strain as a probiotic. Moreover, their viability and stability during production and storage processing are also important issues in the clinical application of probiotics. Resistance in probiotics has been a focus of researchers. A major concern in this area would be the increased risk of transferable drug resistance(s) genes from probiotics to other bacterial population.67 Therefore, it is essential to assess their non-transferable or transferable antibiotic resistance at the genome level. It seems that the use of cell-free supernatants of probiotics can address most of the aforementioned concerns.
Getting reliable enough in vivo and human study results are needed for transferring this treatment strategy in human subjects. In the near future, it would be quite possible to employ the probiotics or their products to develop an innovative safe therapy for biofilm-related infection.
Acknowledgments
This work was financially supported by the Kidney Research Center at Tabriz University of Medical Sciences, Tabriz, Iran (IR.TBZMED.REC.1398.080). The authors wish to express their gratitude to Fatemeh Zununi Vahed for her kind assistance. Keyvan Kheyrolahzadeh and Seyed Mahdi Hosseiniyan Khatibi should be considered first coauthors.
Disclosure
All of the authors declare that there are no personal, commercial, political, and any other potential conflicting interests related to the published paper.
References
1. Bjarnsholt T, Buhlin K, Dufrêne YF, et al. Biofilm formation – what we can learn from recent developments. J Intern Med. 2018;284(4):332–345. doi:10.1111/joim.12782 [PMC free article] [PubMed] [CrossRef] [Google Scholar] 2. Sharahi JY, Azimi T, Shariati A, Safari H, Tehrani MK, Hashemi A. Advanced strategies for combating bacterial biofilms. J Cell Physiol. 2019;234:14689–14708. doi:10.1002/jcp.28225 [PubMed] [CrossRef] [Google Scholar] 3. Mathur H, Field D, Rea MC, Cotter PD, Hill C, Ross RP. Fighting biofilms with lantibiotics and other groups of bacteriocins. NPJ Biofilms Microbiomes. 2018;4:9. doi:10.1038/s41522-018-0053-6 [PMC free article] [PubMed] [CrossRef] [Google Scholar] 4. Iannitti T, Palmieri B. Therapeutical use of probiotic formulations in clinical practice. Clin Nutr. 2010;29(6):701–725. doi:10.1016/j.clnu.2010.05.004 [PMC free article] [PubMed] [CrossRef] [Google Scholar] 5. Sanchez B, Delgado S, Blanco-Miguez A, Lourenco A, Gueimonde M, Margolles A. Probiotics, gut microbiota, and their influence on host health and disease. Mol Nutr Food Res. 2017;61(1):1600240. doi:10.1002/mnfr.201600240 [PubMed] [CrossRef] [Google Scholar] 6. Invernici MM, Salvador SL, Silva PHF, et al. Effects of Bifidobacterium probiotic on the treatment of chronic periodontitis: a randomized clinical trial. J Clin Periodontol. 2018;45(10):1198–1210. doi:10.1111/jcpe.12995 [PMC free article] [PubMed] [CrossRef] [Google Scholar] 7. Chao Y, Zhang T. Surface-enhanced Raman scattering (SERS) revealing chemical variation during biofilm formation: from initial attachment to mature biofilm. Anal Bioanal Chem. 2012;404(5):1465–1475. doi:10.1007/s00216-012-6225-y [PMC free article] [PubMed] [CrossRef] [Google Scholar] 8. Høiby N, Ciofu O, Johansen HK, et al. The clinical impact of bacterial biofilms. Int J Oral Sci. 2011;3(2):55. doi:10.4248/IJOS11026 [PMC free article] [PubMed] [CrossRef] [Google Scholar] 9. Fux C, Costerton JW, Stewart PS, Stoodley P. Survival strategies of infectious biofilms. Trends Microbiol. 2005;13(1):34–40. doi:10.1016/j.tim.2004.11.010 [PubMed] [CrossRef] [Google Scholar] 10. Naves P, Del Prado G, Huelves L, et al. Effects of human serum albumin, ibuprofen and N-acetyl-L-cysteine against biofilm formation by pathogenic Escherichia coli strains. J Hosp Infect. 2010;76(2):165–170. doi:10.1016/j.jhin.2010.05.011 [PubMed] [CrossRef] [Google Scholar] 11. Wu H, Moser C, Wang H-Z, Høiby N, Song Z-J. Strategies for combating bacterial biofilm infections. Int J Oral Sci. 2015;7(1):1–7. doi:10.1038/ijos.2014.65 [PMC free article] [PubMed] [CrossRef] [Google Scholar] 12. Brooks BD, Brooks AE. Therapeutic strategies to combat antibiotic resistance. Adv Drug Deliv Rev. 2014;78:14–27. doi:10.1016/j.addr.2014.10.027 [PubMed] [CrossRef] [Google Scholar] 13. Kinnari TJ. The role of biofilm in chronic laryngitis and in head and neck cancer. Curr Opin Otolaryngol Head Neck Surg. 2015;23(6):448–453. doi:10.1097/MOO.0000000000000200 [PubMed] [CrossRef] [Google Scholar] 14. Hentzer M, Givskov M. Pharmacological inhibition of quorum sensing for the treatment of chronic bacterial infections. J Clin Invest. 2003;112(9):1300–1307. doi:10.1172/jci20074 [PMC free article] [PubMed] [CrossRef] [Google Scholar] 15. Hentzer M, Givskov M, Parsek MR. Targeting quorum sensing for treatment of chronic bacterial biofilm infections. Lab Med. 2002;33(4):295–306. doi:10.1309/EYEV-WT6T-GKHE-C8LM [CrossRef] [Google Scholar] 16. Garcia-Contreras R, Nunez-Lopez L, Jasso-Chavez R, et al. Quorum sensing enhancement of the stress response promotes resistance to quorum quenching and prevents social cheating. ISME J. 2015;9(1):115–125. doi:10.1038/ismej.2014.98 [PMC free article] [PubMed] [CrossRef] [Google Scholar] 17. Bandyopadhyay D, Prashar D, Luk YY. Anti-fouling chemistry of chiral monolayers: enhancing biofilm resistance on racemic surface. Langmuir. 2011;27(10):6124–6131. doi:10.1021/la200230t [PubMed] [CrossRef] [Google Scholar] 18. Speranza B, Liso A, Corbo MR. Use of design of experiments to optimize the production of microbial probiotic biofilms. PeerJ. 2018;6:e4826. doi:10.7717/peerj.4826 [PMC free article] [PubMed] [CrossRef] [Google Scholar] 19. Becirovic A, Abdi-Dezfuli JF, Hansen MF, Lie SA, Vasstrand EN, Bolstad AI. The effects of a probiotic milk drink on bacterial composition in the supra- and subgingival biofilm: a pilot study. Benef Microbes. 2018;1–10. doi:10.3920/bm2018.0009 [PubMed] [CrossRef] [Google Scholar] 20. Lee SH, Kim YJ. A comparative study of the effect of probiotics on cariogenic biofilm model for preventing dental caries. Arch Microbiol. 2014;196(8):601–609. doi:10.1007/s00203-014-0998-7 [PubMed] [CrossRef] [Google Scholar] 21. Brackman G, Coenye T. In vitro and in vivo biofilm wound models and their application. Adv Exp Med Biol. 2016;897:15–32. doi:10.1007/5584_2015_5002 [PubMed] [CrossRef] [Google Scholar] 22. Lkhagvadorj E, Nagata S, Wada M, et al. Anti-infectious activity of synbiotics in a novel mouse model of methicillin-resistant Staphylococcus aureus infection. Microbiol Immunol. 2010;54(5):265–275. doi:10.1111/j.1348-0421.2010.00224.x [PubMed] [CrossRef] [Google Scholar] 23. Jones M, Ganopolsky JG, Labbe A, et al. Novel nitric oxide producing probiotic wound healing patch: preparation and in vivo analysis in a New Zealand white rabbit model of ischaemic and infected wounds. Int Wound J. 2012;9(3):330–343. doi:10.1111/j.1742-481X.2011.00889.x [PubMed] [CrossRef] [Google Scholar] 24. Cortes-Acha B, Figueiredo R, Blanc V, Soler-Olle A, Leon R, Valmaseda-Castellon E. Development and viability of biofilms grown on experimental abutments mimicking dental implants: an in vivo model. Med Oral Patol Oral Cir Bucal. 2019;24(4):e511–e517. doi:10.4317/medoral.22868 [PMC free article] [PubMed] [CrossRef] [Google Scholar] 25. Besser M, Terberger J, Weber L, et al. Impact of probiotics on pathogen survival in an innovative human plasma biofilm model (hpBIOM). J Transl Med. 2019;17(1):243. doi:10.1186/s12967-019-1990-4 [PMC free article] [PubMed] [CrossRef] [Google Scholar] 26. Sharma V, Harjai K, Shukla G. Effect of bacteriocin and exopolysaccharides isolated from probiotic on P. aeruginosa PAO1 biofilm. Folia Microbiol (Praha). 2018;63(2):181–190. doi:10.1007/s12223-017-0545-4 [PubMed] [CrossRef] [Google Scholar] 27. Merghni A, Dallel I, Noumi E, et al. Antioxidant and antiproliferative potential of biosurfactants isolated from Lactobacillus casei and their anti-biofilm effect in oral Staphylococcus aureus strains. Microb Pathog. 2017;104:84–89. doi:10.1016/j.micpath.2017.01.017 [PubMed] [CrossRef] [Google Scholar] 28. Yan X, Gu S, Cui X, et al. Antimicrobial, anti-adhesive and anti-biofilm potential of biosurfactants isolated from Pediococcus acidilactici and Lactobacillus plantarum against Staphylococcus aureus CMCC26003. Microb Pathog. 2019;127:12–20. doi:10.1016/j.micpath.2018.11.039 [PubMed] [CrossRef] [Google Scholar] 29. Kim Y, Kim SH. Released exopolysaccharide (r-EPS) produced from probiotic bacteria reduce biofilm formation of enterohemorrhagic Escherichia coli O157: H7. Biochem Biophys Res Commun. 2009;379(2):324–329. doi:10.1016/j.bbrc.2008.12.053 [PubMed] [CrossRef] [Google Scholar] 30. Pan D, Mei X. Antioxidant activity of an exopolysaccharide purified from Lactococcus lactis subsp. lactis 12. Carbohydr Polym. 2010;80(3):908–914. doi:10.1016/j.carbpol.2010.01.005 [CrossRef] [Google Scholar] 31. Mahdhi A, Leban N, Chakroun I, et al. Extracellular polysaccharide derived from potential probiotic strain with antioxidant and antibacterial activities as a prebiotic agent to control pathogenic bacterial biofilm formation. Microb Pathog. 2017;109:214–220. doi:10.1016/j.micpath.2017.05.046 [PubMed] [CrossRef] [Google Scholar] 32. Kim -N-N, Kim WJ, Kang -S-SJFC. Anti-biofilm effect of crude bacteriocin derived from Lactobacillus brevis DF01 on Escherichia coli and Salmonella Typhimurium. Food Control. 2019;98:274–280. doi:10.1016/j.foodcont.2018.11.004 [CrossRef] [Google Scholar] 33. Okuda K, Zendo T, Sugimoto S, et al. Effects of bacteriocins on methicillin-resistant Staphylococcus aureus biofilm. Antimicrob Agents Chemother. 2013;57(11):5572–5579. doi:10.1128/aac.00888-13 [PMC free article] [PubMed] [CrossRef] [Google Scholar] 34. Algburi A, Volski A, Chikindas ML. Natural antimicrobials subtilosin and lauramide arginine ethyl ester synergize with conventional antibiotics clindamycin and metronidazole against biofilms of Gardnerella vaginalis but not against biofilms of healthy vaginal lactobacilli. Pathog Dis. 2015;73(5):ftv018. doi:10.1093/femspd/ftv018 [PubMed] [CrossRef] [Google Scholar] 35. Turovskiy Y, Cheryian T, Algburi A, et al. Susceptibility of Gardnerella vaginalis biofilms to natural antimicrobials subtilosin, ε-poly-L-lysine, and lauramide arginine ethyl ester. Infect Dis Obstet Gynecol. 2012;2012:284762. doi:10.1155/2012/284762 [PMC free article] [PubMed] [CrossRef] [Google Scholar] 36. Van Kuijk S, Noll K, Chikindas M. The species‐specific mode of action of the antimicrobial peptide subtilosin against Listeria monocytogenes Scott A. Lett Appl Microbiol. 2012;54(1):52–58. doi:10.1111/j.1472-765X.2011.03170.x [PubMed] [CrossRef] [Google Scholar] 37. Algburi A, Zehm S, Netrebov V, Bren AB, Chistyakov V, Chikindas ML. Subtilosin prevents biofilm formation by inhibiting bacterial quorum sensing. Probiotics Antimicrob Proteins. 2017;9(1):81–90. doi:10.1007/s12602-016-9242-x [PubMed] [CrossRef] [Google Scholar] 38. Chopra L, Singh G, Kumar Jena K, Sahoo DK. Sonorensin: a new bacteriocin with potential of an anti-biofilm agent and a food biopreservative. Sci Rep. 2015;5:13412. doi:10.1038/srep13412 [PMC free article] [PubMed] [CrossRef] [Google Scholar] 39. Matsubara VH, Wang Y, Bandara HM, Mayer MP, Samaranayake LP. Probiotic lactobacilli inhibit early stages of Candida albicans biofilm development by reducing their growth, cell adhesion, and filamentation. Appl Microbiol Biotechnol. 2016;100(14):6415–6426. doi:10.1007/s00253-016-7527-3 [PubMed] [CrossRef] [Google Scholar] 40. Zakaria Gomaa E. Antimicrobial and anti-adhesive properties of biosurfactant produced by lactobacilli isolates, biofilm formation and aggregation ability. J Gen Appl Microbiol. 2013;59(6):425–436. doi:10.2323/jgam.59.425 [PubMed] [CrossRef] [Google Scholar] 41. Tan Y, Leonhard M, Moser D, Schneider-Stickler B. Inhibition activity of Lactobacilli supernatant against fungal-bacterial multispecies biofilms on silicone. Microb Pathog. 2017;113:197–201. doi:10.1016/j.micpath.2017.10.051 [PubMed] [CrossRef] [Google Scholar] 42. Wasfi R, Abd El‐Rahman OA, Zafer MM, Ashour HM. Probiotic Lactobacillus sp. inhibit growth, biofilm formation and gene expression of caries‐inducing Streptococcus mutans. J Cell Mol Med. 2018;22(3):1972–1983. doi:10.1111/jcmm.13496 [PMC free article] [PubMed] [CrossRef] [Google Scholar] 43. James KM, MacDonald KW, Chanyi RM, Cadieux PA, Burton JP. Inhibition of Candida albicans biofilm formation and modulation of gene expression by probiotic cells and supernatant. J Med Microbiol. 2016;65(4):328–336. doi:10.1099/jmm.0.000226 [PubMed] [CrossRef] [Google Scholar] 44. Kiymaci ME, Altanlar N, Gumustas M, Ozkan SA, Akin A. Quorum sensing signals and related virulence inhibition of Pseudomonas aeruginosa by a potential probiotic strain’s organic acid. Microb Pathog. 2018;121:190–197. doi:10.1016/j.micpath.2018.05.042 [PubMed] [CrossRef] [Google Scholar] 45. Lopes EG, Moreira DA, Gullon P, Gullon B, Cardelle-Cobas A, Tavaria FK. Topical application of probiotics in skin: adhesion, antimicrobial and antibiofilm in vitro assays. J Appl Microbiol. 2017;122(2):450–461. doi:10.1111/jam.13349 [PubMed] [CrossRef] [Google Scholar] 46. Tahmourespour A, Salehi R, Kermanshahi RK, Eslami G. The anti-biofouling effect of Lactobacillus fermentum-derived biosurfactant against Streptococcus mutans. Biofouling. 2011;27(4):385–392. doi:10.1080/08927014.2011.575458 [PubMed] [CrossRef] [Google Scholar] 47. Jensen PO, Givskov M, Bjarnsholt T, Moser C. The immune system vs. Pseudomonas aeruginosa biofilms. FEMS Immunol Med Microbiol. 2010;59(3):292–305. doi:10.1111/j.1574-695X.2010.00706.x [PubMed] [CrossRef] [Google Scholar] 48. Fong FLY, Shah NP, Kirjavainen P, El-Nezami H. Mechanism of action of probiotic bacteria on intestinal and systemic immunities and antigen-presenting cells. Int Rev Immunol. 2016;35(3):179–188. doi:10.3109/08830185.2015.1096937 [PubMed] [CrossRef] [Google Scholar] 49. Llewellyn A, Foey A. Probiotic modulation of innate cell pathogen sensing and signaling events. Nutrients. 2017;9(10):1156. doi:10.3390/nu9101156 [PMC free article] [PubMed] [CrossRef] [Google Scholar] 50. Dargahi N, Johnson J, Donkor O, Vasiljevic T, Apostolopoulos V. Immunomodulatory effects of Streptococcus thermophilus on U937 monocyte cell cultures. J Functional Foods. 2018;49:241–249. doi:10.1016/j.jff.2018.08.038 [CrossRef] [Google Scholar] 51. Balzaretti S, Taverniti V, Guglielmetti S, et al. A novel rhamnose-rich hetero-exopolysaccharide isolated from Lactobacillus paracasei DG activates THP-1 human monocytic cells. Appl Environ Microbiol. 2017;83(3):e02702–16. doi:10.1128/AEM.02702-16 [PMC free article] [PubMed] [CrossRef] [Google Scholar] 52. Kolenbrander PE, Palmer RJ, Periasamy S, Jakubovics NS. Oral multispecies biofilm development and the key role of cell–cell distance. Nat Rev Microbiol. 2010;8(7):471–480. doi:10.1038/nrmicro2381 [PubMed] [CrossRef] [Google Scholar] 53. Comelli EM, Guggenheim B, Stingele F, Neeser JR. Selection of dairy bacterial strains as probiotics for oral health. Eur J Oral Sci. 2002;110(3):218–224. doi:10.1034/j.1600-0447.2002.21216.x [PubMed] [CrossRef] [Google Scholar] 54. Kaur S, Sharma P, Kalia N, Singh J, Kaur S. Anti-biofilm properties of the fecal probiotic lactobacilli against vibrio spp. Front Cell Infect Microbiol. 2018;8:120. doi:10.3389/fcimb.2018.00120 [PMC free article] [PubMed] [CrossRef] [Google Scholar] 55. Lau CS, Chamberlain RS. Probiotics are effective at preventing Clostridium difficile-associated diarrhea: a systematic review and meta-analysis. Int J Gen Med. 2016;9:27–37. doi:10.2147/IJGM.S98280 [PMC free article] [PubMed] [CrossRef] [Google Scholar] 56. Rumbaugh KP, Griswold JA, Hamood AN. The role of quorum sensing in the in vivo virulence of Pseudomonas aeruginosa. Microbes Infect. 2000;2(14):1721–1731. doi:10.1016/s1286-4579(00)01327-7 [PubMed] [CrossRef] [Google Scholar] 57. Ramos AN, Sesto Cabral ME, Noseda D, Bosch A, Yantorno OM, Valdez JC. Antipathogenic properties of L actobacillus plantarum on P seudomonas aeruginosa: the potential use of its supernatants in the treatment of infected chronic wounds. Wound Repair Regen. 2012;20(4):552–562. doi:10.1111/j.1524-475X.2012.00798.x [PubMed] [CrossRef] [Google Scholar] 58. Varma P, Nisha N, Dinesh KR, Kumar AV, Biswas R. Anti-infective properties of Lactobacillus fermentum against Staphylococcus aureus and Pseudomonas aeruginosa. J Mol Microbiol Biotechnol. 2011;20(3):137–143. doi:10.1159/000328512 [PubMed] [CrossRef] [Google Scholar] 59. Salas-Jara MJ, Ilabaca A, Vega M, Garcia A. Biofilm forming lactobacillus: new challenges for the development of probiotics. Microorganisms. 2016;4(3):35. doi:10.3390/microorganisms4030035 [PMC free article] [PubMed] [CrossRef] [Google Scholar] 60. Gómez NC, Ramiro JM, Quecan BX, de Melo Franco BD. Use of potential probiotic lactic acid bacteria (LAB) biofilms for the control of Listeria monocytogenes, Salmonella Typhimurium, and Escherichia coli O157: H7 biofilms formation. Front Microbiol. 2016;7:863. doi:10.3389/fmicb.2016.00863 [PMC free article] [PubMed] [CrossRef] [Google Scholar] 61. Chen Q, Zhu Z, Wang J, et al. Probiotic E. coli Nissle 1917 biofilms on silicone substrates for bacterial interference against pathogen colonization. Acta Biomater. 2017;50:353–360. doi:10.1016/j.actbio.2017.01.011 [PubMed] [CrossRef] [Google Scholar] 62. Berrios P, Fuentes JA, Salas D, et al. Inhibitory effect of biofilm-forming Lactobacillus kunkeei strains against virulent Pseudomonas aeruginosa in vitro and in honeycomb moth (Galleria mellonella) infection model. Benef Microbes. 2018;9(2):257–268. doi:10.3920/bm2017.0048 [PubMed] [CrossRef] [Google Scholar] 63. Wallis JK, Kromker V, Paduch JH. Biofilm challenge: lactic acid bacteria isolated from bovine udders versus staphylococci. Foods. 2019;8(2). doi:10.3390/foods8020079 [PMC free article] [PubMed] [CrossRef] [Google Scholar] 64. Aoudia N, Rieu A, Briandet R, et al. Biofilms of Lactobacillus plantarum and Lactobacillus fermentum: effect on stress responses, antagonistic effects on pathogen growth and immunomodulatory properties. Food Microbiol. 2016;53(Pt A):51–59. doi:10.1016/j.fm.2015.04.009 [PubMed] [CrossRef] [Google Scholar] 65. Song H, Zhang J, Qu J, et al. Lactobacillus rhamnosus GG microcapsules inhibit Escherichia coli biofilm formation in coculture. Biotechnol Lett. 2019;41:1007–1014. doi:10.1007/s10529-019-02694-2 [PubMed] [CrossRef] [Google Scholar] 66. Kravtsov EG, Yermolayev AV, Anokhina IV, Yashina NV, Chesnokova VL, Dalin MV. Adhesion characteristics of lactobacillus is a criterion of the probiotic choice. Bull Exp Biol Med. 2008;145(2):232–234. doi:10.1007/s10517-008-0058-x [PubMed] [CrossRef] [Google Scholar] 67. Additives EPo, Feed PoSuiA. Guidance on the assessment of bacterial susceptibility to antimicrobials of human and veterinary importance. EFSA J. 2012;10(6):2740. [Google Scholar] 68. Benic GZ, Farella M, Morgan XC, et al. Oral probiotics reduce halitosis in patients wearing orthodontic braces: a randomized, triple-blind, placebo-controlled trial. J Breath Res. 2019;13(3):036010. doi:10.1088/1752-7163/ab1c81 [PubMed] [CrossRef] [Google Scholar] 69. Hallstrom H, Lindgren S, Yucel-Lindberg T, Dahlen G, Renvert S, Twetman S. Effect of probiotic lozenges on inflammatory reactions and oral biofilm during experimental gingivitis. Acta Odontol Scand. 2013;71(3–4):828–833. doi:10.3109/00016357.2012.734406 [PubMed] [CrossRef] [Google Scholar] 70. Lin PP, Hsieh YM, Tsai CC. Isolation and characterisation of probiotics for antagonising the cariogenic bacterium streptococcus mutans and preventing biofilm formation. Oral Health Prev Dent. 2018;16(5):445–455. doi:10.3290/j.ohpd.a41406 [PubMed] [CrossRef] [Google Scholar] 71. Rossoni RD, Velloso MDS, de Barros PP, et al. Inhibitory effect of probiotic Lactobacillus supernatants from the oral cavity on Streptococcus mutans biofilms. Microb Pathog. 2018;123:361–367. doi:10.1016/j.micpath.2018.07.032 [PubMed] [CrossRef] [Google Scholar] 72. Wu CC, Lin CT, Wu CY, Peng WS, Lee MJ, Tsai YC. Inhibitory effect of Lactobacillus salivarius on Streptococcus mutans biofilm formation. Mol Oral Microbiol. 2015;30(1):16–26. doi:10.1111/omi.12063 [PubMed] [CrossRef] [Google Scholar] 73. Krzysciak W, Koscielniak D, Papiez M, et al. Effect of a lactobacillus salivarius probiotic on a double-species streptococcus mutans and candida albicans caries biofilm. Nutrients. 2017;9(11):1242. doi:10.3390/nu9111242 [PMC free article] [PubMed] [CrossRef] [Google Scholar] 74. Rossoni RD, de Barros PP, de Alvarenga JA, et al. Antifungal activity of clinical Lactobacillus strains against Candida albicans biofilms: identification of potential probiotic candidates to prevent oral candidiasis. Biofouling. 2018;34(2):212–225. doi:10.1080/08927014.2018.1425402 [PubMed] [CrossRef] [Google Scholar] 75. Chew SY, Cheah YK, Seow HF, Sandai D, Than LT. In vitro modulation of probiotic bacteria on the biofilm of Candida glabrata. Anaerobe. 2015;34:132–138. doi:10.1016/j.anaerobe.2015.05.009 [PubMed] [CrossRef] [Google Scholar] 76. Keller MK, Hasslof P, Stecksen-Blicks C, Twetman S. Co-aggregation and growth inhibition of probiotic lactobacilli and clinical isolates of mutans streptococci: an in vitro study. Acta Odontol Scand. 2011;69(5):263–268. doi:10.3109/00016357.2011.554863 [PubMed] [CrossRef] [Google Scholar] 77. Lin X, Chen X, Tu Y, Wang S, Chen H. Effect of probiotic lactobacilli on the growth of streptococcus mutans and multispecies biofilms isolated from children with active caries. Med Sci Monit. 2017;23:4175–4181. doi:10.12659/msm.902237 [PMC free article] [PubMed] [CrossRef] [Google Scholar] 78. Jeong D, Kim DH, Song KY, Seo KH. Antimicrobial and anti-biofilm activities of Lactobacillus kefiranofaciens DD2 against oral pathogens. J Oral Microbiol. 2018;10(1):1472985. doi:10.1080/20002297.2018.1472985 [PMC free article] [PubMed] [CrossRef] [Google Scholar] 79. Jiang Q, Stamatova I, Kainulainen V, Korpela R, Meurman JH. Interactions between Lactobacillus rhamnosus GG and oral micro-organisms in an in vitro biofilm model. BMC Microbiol. 2016;16(1):149. doi:10.1186/s12866-016-0759-7 [PMC free article] [PubMed] [CrossRef] [Google Scholar] 80. Jaffar N, Ishikawa Y, Mizuno K, Okinaga T, Maeda T. Mature Biofilm Degradation by Potential Probiotics: aggregatibacter actinomycetemcomitans versus Lactobacillus spp. PLoS One. 2016;11(7):e0159466. doi:10.1371/journal.pone.0159466 [PMC free article] [PubMed] [CrossRef] [Google Scholar] 81. Tan Y, Leonhard M, Moser D, Ma S, Schneider-Stickler B. Inhibitory effect of probiotic lactobacilli supernatants on single and mixed non-albicans Candida species biofilm. Arch Oral Biol. 2018;85:40–45. doi:10.1016/j.archoralbio.2017.10.002 [PubMed] [CrossRef] [Google Scholar] 82. Sarikhani M, Kermanshahi RK, Ghadam P, Gharavi S. The role of probiotic Lactobacillus acidophilus ATCC 4356 bacteriocin on effect of HBsu on planktonic cells and biofilm formation of Bacillus subtilis. Int J Biol Macromol. 2018;115:762–766. doi:10.1016/j.ijbiomac.2018.03.087 [PubMed] [CrossRef] [Google Scholar] 83. Onbas T, Osmanagaoglu O, Kiran F. Potential properties of lactobacillus plantarum F-10 as a bio-control strategy for wound infections. Probiotics Antimicrob Proteins. 2018. doi:10.1007/s12602-018-9486-8 [PubMed] [CrossRef] [Google Scholar] 84. Mahdhi A, Leban N, Chakroun I, et al. Use of extracellular polysaccharides, secreted by Lactobacillus plantarum and Bacillus spp., as reducing indole production agents to control biofilm formation and efflux pumps inhibitor in Escherichia coli. Microb Pathog. 2018;125:448–453. doi:10.1016/j.micpath.2018.10.010 [PubMed] [CrossRef] [Google Scholar] 85. Bidossi A, De Grandi R, Toscano M, et al. Probiotics Streptococcus salivarius 24SMB and Streptococcus oralis 89a interfere with biofilm formation of pathogens of the upper respiratory tract. BMC Infect Dis. 2018;18(1):653. doi:10.1186/s12879-018-3576-9 [PMC free article] [PubMed] [CrossRef] [Google Scholar] 86. Fang K, Jin X, Hong SH. Probiotic Escherichia coli inhibits biofilm formation of pathogenic E. coli via extracellular activity of DegP. Sci Rep. 2018;8(1):4939. doi:10.1038/s41598-018-23180-1 [PMC free article] [PubMed] [CrossRef] [Google Scholar] 87. Melo TA, Dos Santos TF, de Almeida ME, et al. Inhibition of Staphylococcus aureus biofilm by Lactobacillus isolated from fine cocoa. BMC Microbiol. 2016;16(1):250. doi:10.1186/s12866-016-0871-8 [PMC free article] [PubMed] [CrossRef] [Google Scholar] 88. Aarti C, Khusro A, Varghese R, et al. In vitro investigation on probiotic, anti-Candida, and antibiofilm properties of Lactobacillus pentosus strain LAP1. Arch Oral Biol. 2018;89:99–106. doi:10.1016/j.archoralbio.2018.02.014 [PubMed] [CrossRef] [Google Scholar] 89. Kim H, Kang SS. Antifungal activities against Candida albicans, of cell-free supernatants obtained from probiotic Pediococcus acidilactici HW01. Arch Oral Biol. 2019;99:113–119. doi:10.1016/j.archoralbio.2019.01.006 [PubMed] [CrossRef] [Google Scholar] 90. Petrova MI, Imholz NC, Verhoeven TL, et al. Lectin-like molecules of lactobacillus rhamnosus GG inhibit pathogenic escherichia coli and salmonella biofilm formation. PLoS One. 2016;11(8):e0161337. doi:10.1371/journal.pone.0161337 [PMC free article] [PubMed] [CrossRef] [Google Scholar] 91. Jamwal A, Sharma K, Chauhan R, Bansal S, Goel G. Evaluation of commercial probiotic lactic cultures against biofilm formation by Cronobacter sakazakii. Intest Res. 2019;17(2):192–201. doi:10.5217/ir.2018.00106 [PMC free article] [PubMed] [CrossRef] [Google Scholar]